Poly(ε-caprolactone) and polyethylene glycol diacrylate-based scaffolds for TMJ Bioengineered disc implants
Abstract
The temporomandibular joint (TMJ), articulation between the mandibular condyle and the temporal bone, is divided into two compartments (superior and inferior) by a fibrocartilaginous disc. The TMJ disc consists on an avascular and non-innervated tissue, with viscoelastic behavior. Mechanically, the TMJ disc experiences tensile, compressive and shear forces. TMJ disc displacement or degeneration could lead to severe intra-articular temporomandibular joint disorders (TMD). Despite the large number of current therapies/treatments, there is limited treatment options for severe intra-articular TMD. A strategy to solve this problem could be the use of an interposal material to substitute the damaged TMJ disc, which will gradually degrade and give rise to new tissue. In this work three options were used and tested, one using a thermoplastic material, poly(ε-caprolactone) (PCL), another using an photopolymerized hydrogel, polyethylene glycol diacrilate (PEGDA) and the third using the combination of the two materials. Obtained results demonstrated that that the combination of different materials could represent a significant advantage in the TMJ disc TE and in the cartilage TE in general.
Introduction
The temporomandibular joint (TMJ) is a bilateral articulation between the condylar process of the mandible and the mandibular fossa and articular tubercle of the temporal bone. A fibrocartilaginous disc is interposed between the bony structures attached to the fibrous capsule at its margin, which divides the joint into two cavities: superior and inferior.
The TMJ disc consists of an avascular, non-innervated, fibrocartilaginous tissue, with viscoelastic behavior. The disc presents an elliptical shape, longer in the mediolateral direction than in the anteroposterior direction (19 and 13 mm respectively, in humans). Anatomically, the TMJ disc can be divided into three regions: anterior band, intermediate zone and posterior band. The intermediate zone is considerably thinner than the bands (≈1 mm, in humans), and the posterior band is the thickest region of the disc (≈4 mm, in humans). The major fraction of the TMJ disc is composed of water (≈73 %). Apart from water, the TMJ disc is composed of cells (fibroblasts, fibrocytes and chondrocyte-like cells, with a rounded shape and an extracellular matrix (ECM) of dense collagen fibers (≈80 % per dry weight), elastic fibers (≈2 %) and glycosaminoglycans.
This biconcave shape fits on the bone anatomy of the joint and is essential for TMJ functions. As in other joints, TMJ disc reduces friction in the joint and distributes the intra-articular load. Moreover, it helps the joint alignment and stabilization during rotation and translation due to some flexibility provided by the intermediate region. The TMJ disc has a viscoelastic behavior and experiences tensile, compressive and shear forces. Reported compressive properties of the TMJ disc are highly inconsistent, ranging from dozens to tens of thousands of kPa. Regarding different regions, the anterior and posterior bands appear to have higher compressive properties than the intermediate zone.
TMJ disc degeneration and displacement may lead to TMJ disorders (TMD). It is estimated that 25% of the population may experience symptoms of TMD, such as: pain in the joint and in the surrounding muscles; clicks; discomfort when moving the jaw; grind of teeth. The majority of the TMD are successfully treated with non-invasive treatments (e.g.: physical therapy, occlusal splints/adjustments and pharmacology) but, in some cases, it is necessary to resort to surgery (e.g.: arthrocentesis, arthroscopy, discopexy, discectomy and total joint replacement).
The equivalent anatomy to the human TMJ has been studied in different animals. Some of the most common models include pig, sheep, goat, cow, dog, rat and rabbit. In this work, the geometry of the TMJ disc implants was obtained by reverse engineering of the TMJ disc of Black Merino sheep (Ovies aries).
In the last two decades, TMJ disc Tissue Engineering (TE) field has been growing as a promising solution to the TMD, aiming the disc repair and regeneration. Different approaches have been developed, using or not scaffolds, a variety of different materials, and the inclusion of some growth factors.
Scaffolds used in TMJ disc TE has been produced in polyamide, polyglycolic acid (PGA), poly(glycerol sebacate) (PGS), polylactic acid (PLA), poly-L-lactic acid (PLLA), polytetrafluoroethylene (PTFE), and other natural biomaterials, such as collagen hydrogels and from decellularized pig TMJ discs. In the last years, due to their slow rate degradation, poly(ɛ-caprolactone) (PCL) scaffolds and foams have been widely investigated, considering the slow rate of fibrocartilage regeneration.
This work focuses on the development of a PCL and PEGDA-based scaffold for TMJ bioengineered disc implant, with properties close to native tissue, produced with rapid prototyping techniques and feasibly surgical approach.
Materials & Methods
Different scaffolds were produced using reverse engineering and additive manufacturing techniques, in order to replace and promote the regeneration of the TMJ disc ECM. In this investigation three types of 3D matrices were produced: i) PEGDA hydrogel through photopolymerisation, ii) PCL scaffolds using fused deposition modeling (FDM), iii) combination of both.
Poly (ɛ-caprolactone) scaffold production
Poly (ɛ-caprolactone) (PCL) is a biocompatible and biodegradable polyester (12-24 months), which has shown to be capable of supporting a wide variety of cell types and it is a Food and Drug Administration (FDA) approved material. Extrusion process or fused-deposition modeling (FDM) consists on the extrusion of a (thermo)plastic or wax, in this case PCL (as filament or pellets), through a nozzle that draws the pretended built layer-by-layer. Resistive heaters covering the supply channel and the nozzle are used to maintain the material just above its melting point (about 60ºC for PCL). This allows the material to easily flow through the nozzle, bonding to the layer below and achieving prompt solidification.
In the first experiment, the influence of the nozzle temperature on the scaffolds mechanical behavior was investigated. Thus, PCL 6500 (Perstorp) scaffolds (n=4) were produced using the BioExtruder machine (CDRSP, Leiria, Portugal) with a nozzle temperature of 80ºC (group T80) and 86ºC (group T60). In addition, the deposit and runner/gating system temperatures were, respectively, 80ºC and 100ºC, the nozzle used had 300 μm of diameter and the deposition and cross head speeds were 14.5 rpm and 10 mm∙s-1, respectively. The final PCL scaffolds, which its geometry was obtained by reverse engineering of a sheep TMJ disc, had a base of 278 ± 2 mm2 and a slice thickness of 280 μm.
PEGDA hydrogels production
Poly(ethylene glycol), PEG, is a biocompatible, hydrophilic, neutral, and highly mobile polymer chain that has been used in the TE of bone and cartilage, among other biomedical applications, such as controlled drug release systems. Poly(ethylene glycol) diacrylate, PEGDA, is one PEG derivative that is easily manipulated into hydrogels via photopolymerization using biocompatible photoinitiators, such as DMPA (2,2-Dimethoxy-2-phenyl- acetophenone), and exposure to long wavelength UV light, inducing cross-linking.
PEGDA (Sigma-Aldrich) hydrogels (n=4) were produced with two different concentrations, 20% (group H20) and 30%w/V (group H30), with 2-[4-(2-hydroxyethyl) piperazin-1-yl]ethanesulfonic acid (HEPES) buffer (Sigma-Aldrich). Photopolymerization was induced using 0.1% w/V 2,2-dimethoxy-1,2-diphenylethanone (DMPA, Sigma- Aldrich) photoinitiator at UV light (365 nm) exposure. A proper shape of the TMJ disc were obtained through the use of a cutting tool created exclusively for the purpose.
Surface treatment
In order to improve PCL scaffolds hydrophilicity, two main strategies were performed: i) 4 scaffolds (from T86 group) were placed in a sodium hydroxide (NaOH) 1M solution for approximately 24 hours (PNaOH); ii) other 4 scaffolds (from T80 group) were placed in a PEGDA solution before the photopolymerisation (PH).
Mechanical tests
Scaffolds and hydrogels’ mechanical behavior was assessed by uniaxial unconfined compression tests using an universal testing machine with using an extension rate of 1 mm.min-1. Before the mechanical tests, the area of each scaffold was determined using ImageJ2 software. The force, F (N) and deformation (mm) were recorded at any given moment throughout the tests and the compressive stress and strain values were determined to plot the stress-strain graphs. Thus, the elastic compressive modulus was estimated according to the slope of the initial linear region of the plotted curves.
Statistical analysis
Univariate descriptive statistics (mean and standard deviation) of the mechanical behavior were determined for each group. The significant differences between the groups were assessed by one-way ANOVA. Post-hoc tests (Tukey’s test) were conducted to identify any significant differences (p<0.05) for different nozzle temperatures during production and for the superficial treatment, in scaffolds, and for the different PEGDA concentrations in the case of hydrogels. Those tests were performed on GraphPad Prism 6 (GraphPad Software, Inc.) and Excel 2016 (Microsoft Corporation). ImageJ2 (public domain) was used to determine scaffolds and hydrogels areas.
Results & Discussion
Using the Bioextruder we are able to successfully produce reproducible scaffolds (26 x 13 x 4 mm maximum) with the intended geometry obtained through the use of the reverse engineering of a native disc (Fig. 1).
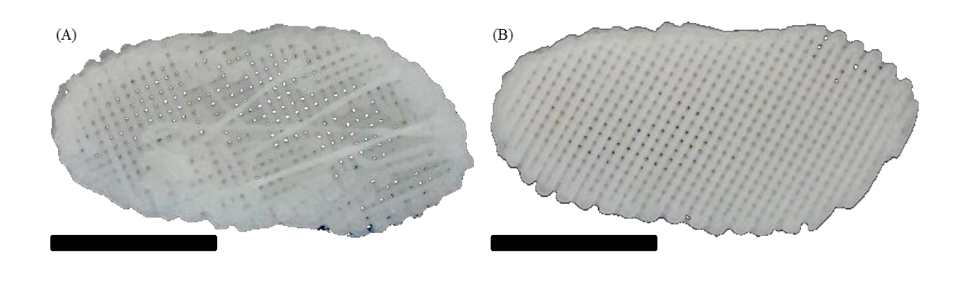
Assessment of the nozzle temperature influence
PCL TMJ disc scaffolds were successfully obtained using the two distinct nozzle temperatures. Results obtained through uniaxial unconfined compression testing indicates that there are significant differences between the two groups (p<0.05) (see Fig. 3). The groups T80 and T86 presented, a compressive modulus of 31.8 ± 1.5 MPa and 23.9 ± 1.8 MPa, respectively.
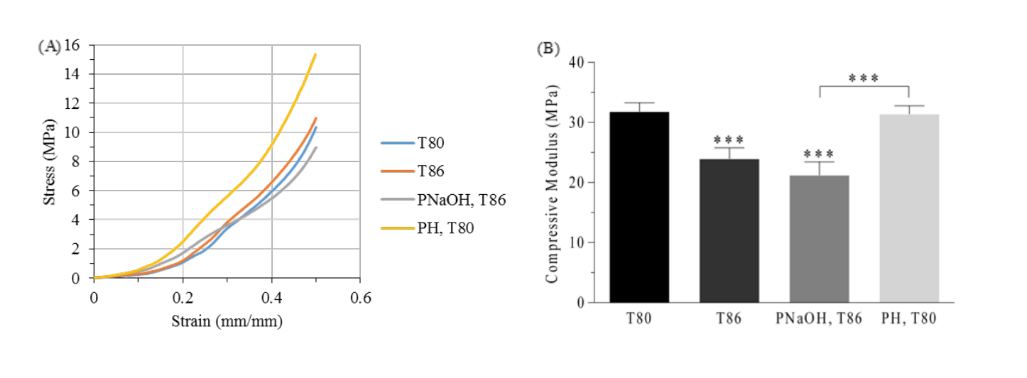
According to the literature the temperature directly influence the road width, influencing the final porosity (higher temperature leads to a lower porosity) of the structures, and consequently its mechanical behavior. A lower temperature allows the material to solidify faster reducing possible changes in scaffolds geometry. Those changes can occur at higher production temperatures because the material, being in a viscous state, is affected by gravity and the scaffolds become slightly flat. This experiment is in concordance with the expected result.
Assessment of the influence of PEGDA concentration on the mechanical properties of hydrogels
As shown in figure 2, significant differences are presented between the two groups of hydrogels (p<0.05). The hydrogels produced with a PEGDA concentration of 20% w/V presented almost half of the compressive modulus and a third of the ultimate strength (1.30 ± 0.15 MPa and 0.30 ± 0.03 MPa, respectively) when compared to the hydrogels produced with 30% w/V of PEGDA (2.21 ± 0.16 MPa and 1.01 ± 0.11 MPa). Despite of that, the hydrogels of the group H30 showed to be highly brittle when bending or pulling. Thus, to produce the scaffolds of the group PH, hydrogels similar to the group P20 were used.
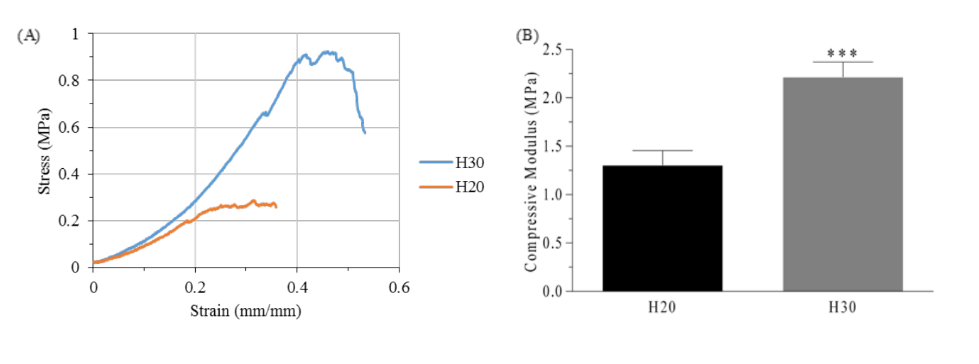
Influence of surface treatment
The surface treatment of the PCL scaffolds did not conducted to significant differences in the scaffolds’ mechanical behavior. The compressive modulus of the PNaOH group (21.2 ± 2.2 MPa) slightly decreased compared to the control group (T86), but these differences were not significant (p>0.05). The slightly decrease in mechanical properties is due to the surface degradation promoted by the NaOH solution - decrease in the diameter of the filaments becoming irregular along the fiber. Thus, the scaffolds lose mass and their porosity increases, leading to a decrease of its mechanical properties to compression.
On the other hand, the addition of hydrogel in the group PH did not cause significant differences on the compressive elastic moduli (31.4 ± 1.4 MPa) compared with the control group (T80). Besides, the hydrogel appears to increase significantly the lubrication and flexibility of the scaffolds and it could help in the cellular adhesion, due to the increase of its hydrophilicity.
The use of PCL in cartilage TE, specifically in the fibrocartilaginous tissue of the TMJ disc, is recent. Although the original hydrophobicity of PCL, the surface treatment with NaOH may improve its hydrophilicity and cellular adhesion due to surface irregularity. Moreover, the incorporation of PEGDA hydrogel is an avant-garde approach and it could point the right direction for a successful TMJ bioengineered disc implant. The combination of two different biomaterials (and associated production technologies) may result in significant benefits in the TMJ disc TE. In this approach, PCL provides the necessary mechanical resistance and its slow degradation rate is compatible with the slow growth rate of fibrocartilaginous tissue, while the PEGDA hydrogel promotes cellular adhesion, increase the implant lubrication and allows the integration of growth factors. Although PCL presents a higher stiffness than native TMJ disc tissue, this could be beneficial in surgery, since it allows an easier placement of the implant, reducing time and permitting a less invasive surgery.
Conclusion
The TMJ disc TE aims to offer an alternative to the current treatments of TMD due to disc degeneration. Thus, combining different materials is possible to produce a bioengineered implant with a better mimicking the properties of the native tissue.
In this study, we successfully produced a PCL and PEGDA-based scaffold for TMJ bioengineered disc implant. Due to the slow degradation rate of PCL, this material is compatible with the slow growth rate of TMJ disc tissue. Moreover, PCL provides important mechanical properties to the scaffold and its relative rigidity could represent an advantage during surgery. On the other hand, PEGDA hydrogel promotes cellular adhesion and provides lubrication. Thus, the TMJ disc functions are assured: i) the lubrication allow a reduction of friction in the joint; ii) the distribution of the intra-articular load is assured by the scaffold geometry and by the mechanical properties of the materials; ii) the implant geometry allows joint alignment and stabilization.
In summary, this work demonstrated that the combination of different materials could represent a significant progress in the TMJ disc TE and in the cartilage TE in general.
Authors: Luís Francisco, Carla Moura, Tânia Viana, David Ângelo, Pedro Morouço, Nuno Alves
References:
- VanPutte, C., Regan, J., Russo, A., Seeley, R., Stephens, T. and Tate, P., Seeley's Anatomy & Physiology, 10th Edition, New York, USA, 2014
- Athanasiou, K. A., Almarza, A. A., Detamore, M. S. and Kalpakci, K. N., Tissue Engineering of Temporomandibular Joint Cartilage, 2009
- Stankovic, S., Vlajkovic, S., Boskovic, M., Radenkovic, G., Antic, V. and Jevremovic, D., Morphological and biomechanical features of the temporomandibular joint disc: an overview of recent findings, Arch Oral Biol, 58 (2013) 1475-82
- Kalpakci, K. N., Willard, V. P., Wong, M. E. and Athanasiou, K. A., An interspecies comparison of the temporomandibular joint disc, J Dent Res, 90 (2011) 193-8
- Matuska, A. M., Muller, S., Dolwick, M. F. and McFetridge, P. S., Biomechanical and biochemical outcomes of porcine temporomandibular joint disc deformation, Arch Oral Biol, 64 (2016) 72-9
- Fazaeli, S., Ghazanfari, S., Everts, V., Smit, T. H. and Koolstra, J. H., The contribution of collagen fibers to the mechanical compressive properties of the temporomandibular joint disc, Osteoarthritis Cartilage, 24 (2016) 1292- 301
- Kuo, J., Zhang, L., Bacro, T. and Yao, H., The region-dependent biphasic viscoelastic properties of human temporomandibular joint discs under confined compression, J Biomech, 43 (2010) 1316-21
- Tanaka, E. and Eijden, T. v., Biomechanical behavior of the temporomandibular joint disc, Crit Rev Oral Biol Med, 14 (2003) 138-150
- Beek, M., Aarnts, M. P., Koolstra, J. H., Feilzer, A. J. and Eijden, T. M. G. J. v., Dynamic Properties of the Human Temporomandibular Joint Disc, J Dent Res, 80 (2001) 876-880
- Willard, V. P., Kalpakci, K. N., Reimer, A. J. and Athanasiou, K. A., The regional contribution of glycosaminoglycans to temporomandibular joint disc compressive properties, J Biomech Eng, 134 (2012) 011011
- Ingawale, S. and Goswami, T., Temporomandibular joint: disorders, treatments, and biomechanics, Ann Biomed Eng, 37 (2009) 976-96
- Murphy, M. K., MacBarb, R. F., Wong, M. E., A., K. and Athanasiou, K. A., Temporomandibular Joint Disorders: A Review of Etiology, Clinical Management, and Tissue Engineering Strategies, Int J Oral Maxillofac Implants, 28 (2013) e393–e414
- Herring, S. W., TMJ anatomy and animal models, J Musculoskelet Neuronal Interact, 3 (2003) 391
- Bermejo, A., González, O. and González, J. M., The pig as an animal model for experimentation on the temporomandibular articular complex, Oral Surc Oral Med Oral Pathol, 75 (1993) 18-23
- Angelo, D. F., Morouco, P., Alves, N., Viana, T., Santos, F., Gonzalez, R., Monje, F., Macias, D., Carrapico, B., Sousa, R., Cavaco-Goncalves, S., Salvado, F., Peleteiro, C. and Pinho, M., Choosing sheep (Ovis aries) as animal model for temporomandibular joint research: Morphological, histological and biomechanical characterization of the joint disc, Morphologie, 100 (2016) 223-233
- Mehrotra, D., TMJ Bioengineering: A review, J Oral Biol Craniofac Res, 3 (2013) 140-5
- Hunter, C. J., Fibrocartilage Tissue Engineering, in: J. A. Burdick and R. L. Mauck (Eds.), Biomaterials for Tissue Engineering Applications: A Review of the Past and Future Trends, Springer, NewYork, 2011, pp. 363-389
- Allen, K. D. and Athanasiou, K. A., Scaffold and Growth Factor Selection in Temporomandibular Joint Disc Engineering, J Dent Res, 87 (2008) 180-185
- Juran, C. M., Dolwick, M. F. and McFetridge, P. S., Engineered microporosity: enhancing the early regenerative potential of decellularized temporomandibular joint discs, Tissue Eng Part A, 21 (2015) 829-39
- Springer, I. N. G., Fleiner, B., Jepsen, S. and Açil, Y., Culture of cells gained from temporomandibular joint cartilage on non-absorbable scaffold, Biomaterials, 22 (2001) 2569–2577
- Hagandora, C. K., Gao, J., Wang, Y. and Almarza, A. J., Poly (glycerol sebacate): a novel scaffold material for temporomandibular joint disc engineering, Tissue Eng Part A, 19 (2013) 729-37
- Brown, B. N., Chung, W. L., Almarza, A. J., Pavlick, M. D., Reppas, S. N., Ochs, M. W., Russell, A. J. and Badylak, S. F., Inductive, scaffold-based, regenerative medicine approach to reconstruction of the temporomandibular joint disk, J Oral Maxillofac Surg, 70 (2012) 2656-68
- Kobayashi, E., Nakahara, T., Inoue, M., Shigeno, K., Tanaka, A. and Nakamura, T., Experimental Study on In Situ Tissue Engineering of the Temporomandibular Joint Disc using Autologous Bone Marrow and Collagen Sponge Scaffold, Journal of Hard Tissue Biology, 24 (2015) 211 -218
- Olubamiji, A. D., Izadifar, Z., Si, J. L., Cooper, D. M., Eames, B. F. and Chen, D. X., Modulating mechanical behaviour of 3D-printed cartilage-mimetic PCL scaffolds: influence of molecular weight and pore geometry, Biofabrication, 8 (2016) 025020
- Legemate, K., Tarafder, S., Jun, Y. and Lee, C. H., Engineering Human TMJ Discs with Protein-Releasing 3D-Printed Scaffolds, J Dent Res, 95 (2016) 800-7
- Ratner, B. D., Hofffman, A. S., Schoen, F. J. and Lemons, J. E., Blomaterials Science - An Introduction to Materials in Medicine, San Diego, California, 1996
- Ebnesajjad, S., Handbook of Biopolymers and Biodegradable Plastics - Properties, Processing and Applications, UK, 2013
- Domingos, M., Chiellini, F., Gloria, A., Ambrosio, L., Bartolo, P. and Chiellini, E., Effect of process parameters on the morphological and mechanical properties of 3D Bioextruded poly(ε‐caprolactone) scaffolds, Rapid Prototyping Journal, 18 (2012) 56-67
- Sun, H., Mei, L., Song, C., Cui, X. and Wang, P., The in vivo degradation, absorption and excretion of PCL- based implant, Biomaterials, 27 (2006) 1735-40
To continue learning and get access to all the other articles, log in or create an account